Research
Our lab is
part of the Clean Technologies Research Institute (CTRI) at Dalhousie
University. For more
details about CTRI, see here.
Overall Aims of Our
Research Program
-
to
use a chemical approach to
understand the underlying physical principles relating structure to
properties
of materials;
-
to contribute to the advancement of understanding of
thermal properties of materials;
-
to contribute to basic
understanding of materials, with an eye to applications such as
phase change materials for energy storage and thermal regulation,
thermoelectrics, materials with zero thermal
expansion for reduced thermal stress, and thermochromic materials for
thermally erasable toner.
Selected Current/Recent Research
Our publications are listed
here.
Phase Change Materials:
Thermal Energy Storage and Temperature Regulation
-
Transitions of phase-change materials (PCMs)
can be used for heat storage;
-
PCMs store more energy stored per unit volume or unit mass than
materials that store thermal energy just by heat capacity (sensible
heat);
-
Our goals are to develop materials that store energy very efficiently,
with regard to robust properties after thermal cycling, and
form-stable PCMs with enhanced thermal conductivity.
Dodecanoic acid as a promising phase-change material for
thermal energy storage. L. Desgrosseilliers, C. A. Whitman, D.
Groulx and M. A. White, Appl. Thermal Eng. (2013). |
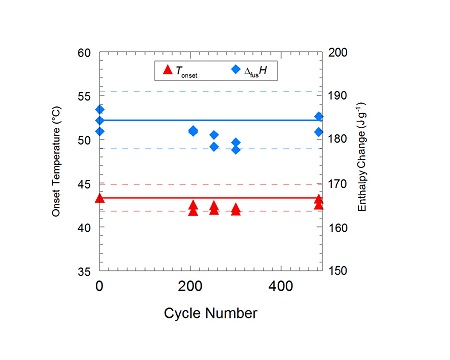 |
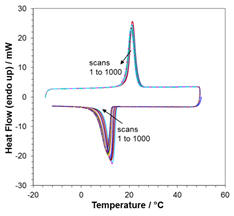 |
Characterization of Thermal Performance of a Solid-Solid Phase
Change Material, Di-n-Hexylammonium Bromide, for Potential
Integration in Building Materials. C. A. Whitman, M.B. Johnson and
M.A. White. Thermochimica Acta
531, 54-59 (2012). |
See also: Edible Oils as
Practical Phase Change Materials for Thermal Energy Storage. S. Kahwaji
and M. A. White. Appl. Sci. 19, 1627:1-17
(2019). Open Access
Heat Transport in
Nanostructured Materials
-
Properties of materials change as they get very small;
-
Heat
conduction is changed by interfaces and boundaries separated by less than
the phonon mean free path;
-
Thermal conductivity is reduced so
Fourier’s Law no longer applies;
-
Nanostructuring is a potentially
useful mechanism to reduce thermal conductivity;
-
Our goal is to understand the thermal conductivity of materials with
micro- to nanoscale level structure, including carbon nanotube materials,
carbon nanotube/polymer composites and complex, natural and synthetic
nanostructures, such as ivory, nacre and synthetic nacre.
Elephant Ivory: a
Low Thermal Conductivity, High Strength Nanocomposite. M.B.
Jakubinek, C. Samarasekera and M.A. White,
Journal of Materials Research,
21,
287-292 (2006).
|
|
|
Relationship
Between Thermal Conductivity and Structure of Nacre from
Haliotis fulgens. L. P. Tremblay, M.B. Johnson, U.
Werner-Zwanziger and M.A. White, Journal of Materials Research26,
1216-1224 (2011).
|
Thermal
conductivity of tunable lamellar Al2O3/PMMA
hybrid composites. R. Chen, M.B. Johnson, K.P. Plucknett and M.A.
White.
J. Mater. Res.
27, 1869-1876 (2012).
|
|
|
Physical properties of sheet drawn from arrays of multiwalled
carbon nanotubes. J.-H. Pöhls, M.B. Johnson, M.A. White, R. Malik,
B. Ruff, C. Jayasinghe, M.J. Schulz and V. Shanov. Carbon
50, 4175-4183 (2012).
See also Jakubinek
et al., Carbon
50, 244-248 (2012), and
Carbon 48,
3947-3952 (2010). |
Controlled Thermal Expansion
-
Most materials expand when
heated, but some, such as ZrW2O8, contract when
heated!
-
Controlled thermal expansion can reduce the thermal stress experienced
by a material when it is exposed to a large temperature gradient;
-
Our goals are to develop materials with low overall thermal expansion,
by preparation of solid solutions of positive and negative thermal
expansion materials, and by manipulating mechanical properties to make
composites with reduced thermal stress;
-
We
also investigate the role of structure on thermal conductivity of
framework materials with low positive (or negative) thermal expansion.
|
The Effect of Microstructure on Thermal Expansion Coefficients
in Powder-Processed
Al2Mo3O12.
L. P.
Prisco, C.P. Romao, F. Rizzo, M.A. White and B. A. Marinkovic,
J. Mater. Sci. (2013).
|
Near-Zero Thermal Expansion in
In(HfMg)0.5Mo3O12.
K.J. Miller, C. P. Romao, M. Bieringer, B. A.
Marinkovic, L.Prisco and M. A. White, J. Amer.
Ceram. Soc. (2013).
|
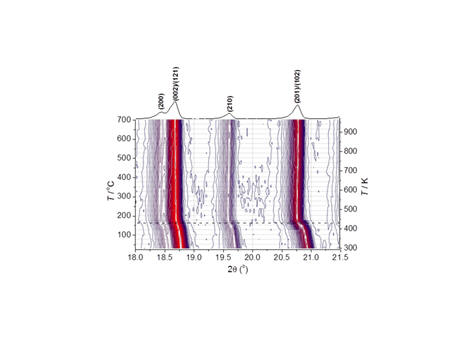 |
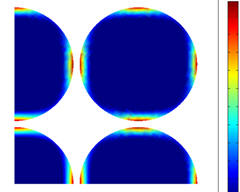 |
Negative Thermal Expansion Materials: Thermal Properties and
Implications for Composite Materials. M. B. Jakubinek, C. A.
Whitman and M. A. White, Journal of Thermal Analysis and
Calorimetry 99(1), 165-172 (2010).
|
Thermoelectric Materials
-
Thermoelectrics convert waste
energy to power, but are not yet highly efficient;
-
Efficiency can be increased by lowering thermal conductivity;
-
Our goal is to understand mechanisms to reduce thermal conductivity,
such as scattering of heat-carrying acoustic phonons by guests
rattling in cage structures.
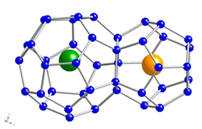 |
X-ray
Absorption Studies of Local Structure and Electronic Properties of
NaxSi136 (0<x<24) Clathrates.
A.D. Ritchie, M.A. MacDonald, P. Zhang, M.A. White, M. Beekman, J.
Gryko and G. Nolas, Phys.Rev.B, 82, 155207:1-9
(2010).
|
Thermal and lattice dynamical properties of Na8Si46
clathrate. L. Qiu, M.A.White, Z. Li, J.S. Tse, C.I. Ratcliffe,
C.A. Tulk, J. Dong and O.F. Sankey, Phys. Rev. B
64, 24303:1-6 (2001). |
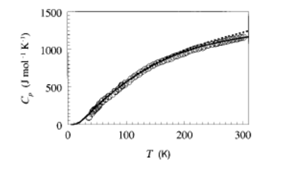 |
Thermochromic Materials
-
Materials that change colour with temperature are thermochromic;
-
We
concentrate on three-component organic systems in which one component
melts, and other two undergo interactions that change the colour;
-
Our aim is to understand the role of packing interactions between
components, and how this affects colour density and staying power.
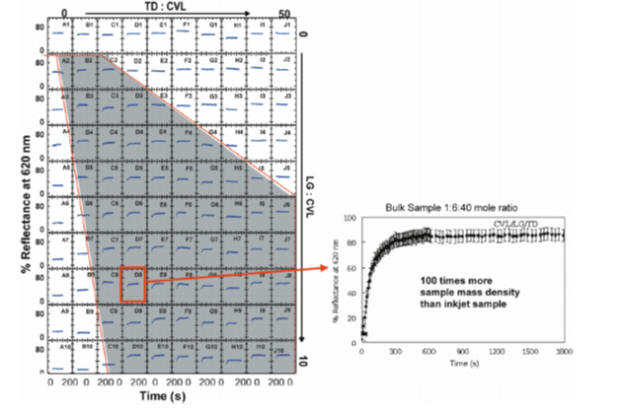 |
New Insights Concerning the Mechanism of Reversible Thermochromic
Mixtures. H. Tang, D. C. MacLaren and M.A. White, Can. J.
Chem.88, 1063-1070 (2010).
|
Current or Recent Research Collaborations
D. L. Bryce, University of Ottawa: NMR Crystallography;
U. Werner-Zwanziger, Dalhousie University: NMR Studies of Materials;
A. Windle, University of Cambridge: Carbon Nanotube Materials;
J. Zwanziger, Dalhousie University: Negative Thermal Expansion Materials;
M. Bieringer,
University of Manitoba: Thermal
Expansion;
D. Groulx,
Dalhousie, Mechanical Engineering: Heat Storage Materials;
J. Majzlan,
Friedrich‑Schiller University, Germany: Mineral Thermodynamics;
B. Marinkovic,
Pont.Univ.Católica do Rio de Janeiro, Brazil: Negative Thermal
Expansion Materials;
G. Nolas,
University of South Florida, USA: Thermoelectric Materials;
B. Simard,
Steacie Institute for Molecular Sciences, NRC, Ottawa: Carbon Nanotube
Composites;
V.
Shanov,
University of Cincinnati, USA: Carbon Nanotube Materials;
A. Wilkinson,
Georgia Tech, USA: Negative Thermal Expansion Materials
|